从 Knoppix 到 OCI-Image-v2
众所周知,Docker 始于2013年的 dotCloud,迄今刚刚七年,如果你刚好在圈中经历了2013-2015年这段早期岁月的话,自然应该知道,最初的 Docker = LXC + aufs,前者就是所谓的 Linux 容器了,而后者则是我今天要聊的镜像。
千禧年:惊艳的 Live CD
说到 Linux distro,除了做差异化的界面主题之外,核心差异一般都在于:
- 如何更方便地安装;
- 如何更方便地升级;
而在 distro 界却有一股清流,超脱于这两件事情之外,它们就是 Live CD,它们装在一张光盘里,或者是一个 U盘上,不需要安装、也不会改变。之前创业的时候,我司的运维大佬——彤哥曾经说过:
第一次见到 liveCD 时我内心是震惊的。。
这我当然是赞同的,那时我也是震惊的同学之一,要知道 Knoppix 在 2000 千禧年就来到了世界,而它所基于的著名的 Debian,直到2005年6月,Sarge (3.1) 发布的时候才正式在 stable release 里带上了图形界面的安装程序 debian-installer (简称 d-i),此前版本的安装还在用文本菜单。就在这个年代,这样一个开光盘即用、启动起来就是图形界面的系统,给我们这些玩家带来的震撼,当然是可想而知的。那时候的 Live CD 就是十三年后的 Docker,绝对配得上“惊艳”两个字。
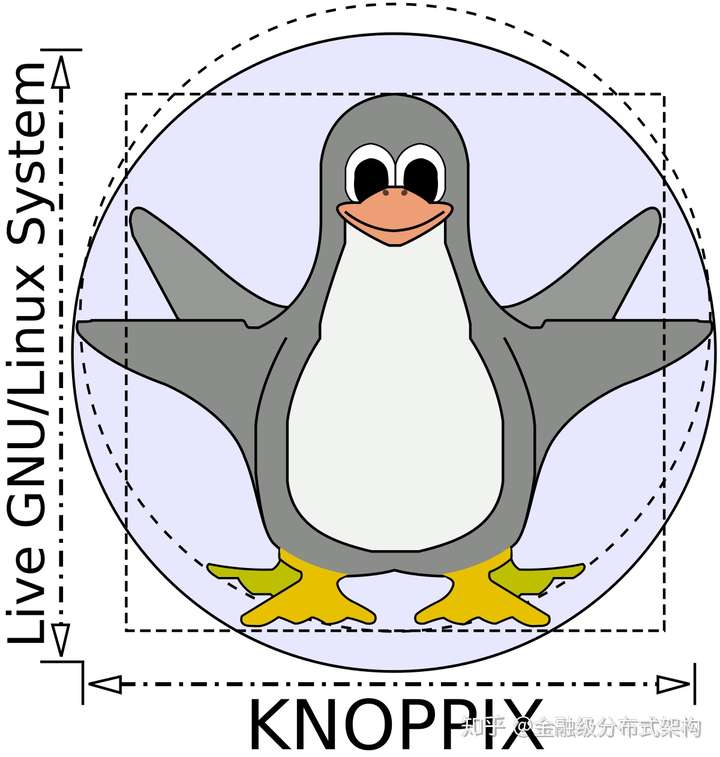
要知道,一张 700MB 左右的光盘里塞下个完整的操作系统并不容易(当然有人开头之后也不太难,后来我爱的 DSL 可以做到 50MB)。Knoppix 有个很棒的想法——把装好的操作系统给压缩一下,放在光盘里, 随用随解压,这样,一张 700MB 光盘里可以放下大约 2GB 的根文件系统,这样就跑 KDE 桌面也就没啥问题了,当是时,http://distrowatch.com 上可以看到,一大片 distro 都是基于 Knoppix 魔改的,足见其影响力。
进化:可读写层与 UnionFS
Knoppix 在诞生之初的一个执念是“绝不碰本地存储一根指头”,而光盘,CD-ROM,所使用的 ISO9600 文件系统也是只读的,这无疑暗合了当今流行的“不可变基础设施”的潮流,但是,即使在今天,没有可写文件系统对于很多 Linux 软件仍然是非常困难的,毕竟随随便便一个程序也要写一点配置文件、状态信息、锁、日志之类的嘛。而诞生之初的 Knoppix 是不可写的,那么,要有什么东西要罗盘,就得手工挖掘出本地硬盘来挂上,或者是挂个 NAS 到 /home 或其他挂载点上来,当你不想只是做个紧急启动盘的时候,这就有点麻烦了。
如果我们从今天穿越回去,毫不费力就可以指出,用 overlayfs 加上一个 tmpfs 做可写层嘛。但是,overlayfs 要到2010年才首次提交 patchset,2014年才被合并到 3.18内核(这中间,当时的淘宝内核组也有不少贡献和踩坑呢)。当然,比 overlay 早的类似的 unionfs 还是有的,Docker 最早采用的 Aufs 就是其中之一,它是2006年出现的,这里 AUFS 的 A,可以理解成 Advanced,但它最早的意思实际是 Another——是的,“另一个 UFS”,而它的前身就是 UnionFS。
在2005年5月,也就是十五年前,Knoppix 创造性地引入了 UnionFS,而在一年半以后的 5.1 版本中,Knoppix 引入了当年诞生的更稳定的 aufs,此后,包括大家熟悉的 Ubuntu LiveCD、Gentoo LiveCD 全都使用了 aufs。可以说,正是 Live CD 们,提前了8年,为 Docker 和 Docker Image 的诞生,做好了存储上的准备。
这里简单说一句给不了解的人听,所谓 union fs,是指多个不同文件系统联合(堆叠)在一起,呈现为一个文件系统,它和一般的 FHS 规定的那种树装组织方式是不同的,如下图,对于左边的标准的目录树结构,任何一个文件系统,挂载在树上的一个挂载点,根据路径,就可以指到一个确定的文件系统,比如,下图中,所有的 /usr/local/
下面的路径都在一个文件系统上,而其他 /usr
就会在另一个文件系统上;而 UnionFS 是多层堆叠的,你写的文件会停留在最上层,比如图中,你修改过的 /etc/passwd
就会在最上的可写层,其他的文件就要去下面的层中去找,也就是说,它允许同一个目录中的不同文件在不同层中,这样,Live CD 操作系统跑起来就像真正的本地操作系统一样可以读写所有路径了。
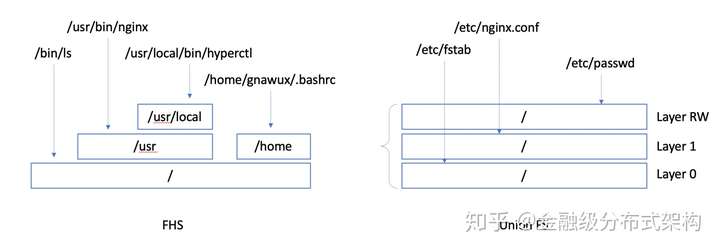
块或文件:Cloop 与 SquashFS
让我们把目光放在只读层上,这一层是 Live CD 的基础,在 Live CD 还没有 union FS 来做分层的时候就已经存在这个只读 rootfs 了。对 Knoppix 来说,这一层是不能直接放完整、无压缩的操作系统的,因为在21世纪初,大家都还在用 24x 到 40x 速光驱的时代,Knoppix Live CD 面临的一个大问题是 700MB 的光盘和庞大的桌面操作系统之间的矛盾。
开头我们提到了,Knoppix 的想法就是“把装好的操作系统给压缩一下,放在光盘里, 随用随解压”,这样精心选择后的 2GB 的 Distro 就可以压到一张光盘里了,不过“随用随解压“不是说有就有的,文件系统访问块设备,是要找到块的偏移量的,压缩了之后,这个偏移量就并不那么好找了,全解压到内存里再找偏移并不那么容易。
回到2000年,那时候还是2.2内核,Knoppix 的作者 Klaus Knopper 在创立 Knoppix 之初就引入了一种压缩的(compressed) loop 设备,称为 cloop,这种设备是一种特殊的格式,它包含了一个索引,从而让解压缩的过程对用户来说是透明的,Knoppix 的 cloop 设备看起来就是一个大约 2GB 大的块设备,当应用读写一个偏移的数据的时候,它只需要根据索引解压缩对应的数据块,并返回数据给用户就可以了,无需全盘解压缩。
尽管 Knoppix 把众多 distro 带上了 Live CD 的船,但是,众多后继者,诸如 arch、Debian、Fedora、Gentoo、Ubuntu 等等 distro 的 LiveCD,以及大家熟悉的路由器上玩的 OpenWrt,都并没有选择 cloop 文件,它们选择了和应用语义更接近的文件系统级的解决方案——Squashfs。Squashfs 压缩了文件、inode 和目录,并支持从 4K 到 1M 的压缩单元尺寸。同样,它也是根据索引按需解压的,和 cloop 的不同之处是,当用户访问一个文件的时候,它来根据索引解压相应的文件所在的块,而非再经过一层本地文件系统到压缩块的寻址,更加简单直接。事实上,Knoppix 里也一直有呼声想要切换到 squashfs,比如,2004年就有开发者在转换 knoppix 到squashfs 上,而且,一些测试数据似乎也表明 Squashfs 的性能似乎要更好一些,尤其在元数据操作方面。
The design of the cloop driver requires that compressed blocks be read whole from disk. This makes cloop access inherently slower when there are many scattered reads, which can happen if the system is low on memory or when a large program with many shared libraries is starting. A big issue is the seek time for CD-ROM drives (~80 ms), which exceeds that of hard disks (~10 ms) by a large factor. On the other hand, because files are packed together, reading a compressed block may thus bring in more than one file into the cache. The effects of tail packing are known to improve seek times (cf. reiserfs, btrfs), especially for small files. Some performance tests related to cloop have been conducted.
我来画蛇添足地翻译一下:
cloop 的设计要求从磁盘上以压缩块为单位读取数据。这样,当有很多随机的读操作时,cloop 就会显著地变慢,当系统内存不足或者一个有很多共享库的大型程序启动的时候都很可能发生。cloop 面临的一个很大的问题是 CD-ROM 的寻道时间(约80毫秒),这在很大程度上超过了硬盘的查找时间(约10毫秒)。另一方面,由于文件可以被打包在一起,因此读取压缩块实际可能可以将多个文件带入缓存。这样,那些支持 tail packing 的文件系统(比如 reiserfs,btrfs)可能可以显著改善 seek 操作的时间,尤其是对于小文件更是如此。已经有一些与 cloop 相关的性能测试也证明了这些观点。
当然,尽管有这些争论,cloop 也仍然在 Knoppix 上存在,不过,这个争论最终随着2009年 squashfs 被并入 2.6.29 主线内核,应该算是分出胜负了,进入 kernel 带来的开箱即用换来的是压倒性的占有率和更好的支持,Squashfs 的优势不仅在上述的 distro 用户之多,也在于支持了了各种的压缩算法,只用于不同的场景。
Docker: Make Unionfs Great Again
斗转星移,不再年轻的 Live CD 也因为如此普及,而让人觉得并不新奇了。但是,技术圈也有轮回一般,当年被 Live CD 带红的 Union FS 们再一次被 Docker 捧红,焕发了第二春。一般地说,虽然 aufs 们支持多个只读层,但普通的 Live CD 只要一个只读镜像和一个可写层留给用户就可以了。然而, 以 Solomon 为首的 dotCloud 的朋友们充分发挥了他们卓越的想象力,把整个文件系统变成了“软件包”的基本单位,从而做到了 #MUGA (Make Unionfs Great Again)。
回想一下,从1993年的 Slackware 到今天的 RHEL,(服务端的)Distro 的所作所为,不外乎我开头提到的两件事情——安装和升级。从 rpm 到 APT/deb 再到 Snappy,初始化好系统之后的工作的精髓就在于怎么更平滑地安装和升级、确保没有依赖问题,又不额外占据太多的空间。解决这个问题的基础就是 rpm/deb 这样的包以及包之间的依赖关系,然而,类似“A 依赖 B 和 C,但 B 却和 C 冲突” 这样的事情仍然层出不穷,让人们不停地尝试解决了二十年。
但 Docker 跳出了软件包这个思路,他们是这么看的——
- 完整的操作系统就是一个包,它必然是自包含的,而且如果在开发、测试、部署时都保持同样完整、不变的操作系统环境,那么也就没有那么多依赖性导致的问题了;
- 这个完整的操作系统都是不可变的,就像 Live CD 一样,我们叫它镜像,可以用 aufs 这样的 union FS 在上面放一个可写层,应用可以在运行时写东西到可写层,一些动态生成的配置也可以放在可写层;
- 如果一些应用软件镜像,它们共用同一部分基础系统,那么,把这些公共部分,放在 Unionfs 的下层作为只读层,这样他们可以给不同的应用使用;当然,如果两个应用依赖的东西不一样,那它们就用不同的基础层,也不需要互相迁就了,自然没有上面的依赖性矛盾存在了;
- 一个镜像可以包含多个层,这样,更方便应用可以共享一些数据,节省存储和传输开销;
大概的示意图是这样的:
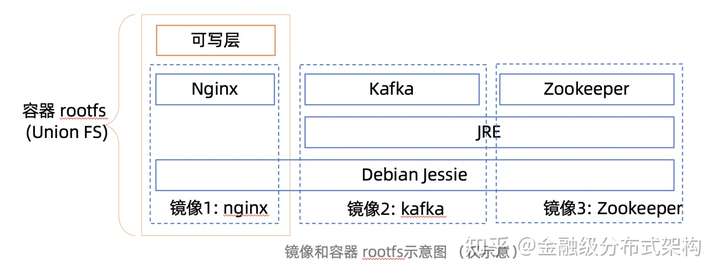
这样,如果在同一台机器上跑这三个应用(容器),那么这些共享的只读层都不需要重复下载。
更进一步说,Docker 这种分层结构的另一个优点在于,它本身是非常开发者友好的,可以看到,下面这个是一个 Dockerfile 的示意,FROM 代表最底下的基础层,之后的 RUN, ADD 这样改变 rootfs 的操作,都会将结果存成一个新的中间层,最终形成一个镜像。这样,开发者对于软件依赖性的组织可以非常清晰地展现在镜像的分层关系中,比如下面这个 Dockerfile 是一个 packaging 用的 image,它先装了软件的依赖包、语言环境,然后初始化了打包操作的用户环境,然后拉源代码,最后把制作软件包的脚本放到镜像里。这个组织方式是从通用到特定任务的组织方式,镜像制作者希望让这些层可以尽量通用一些,底层的内容可以在其他镜像中也用得上,而上层则是和本镜像的工作最直接相关的内容,其他开发者在看到这个 Dockerfile 的时候已经可以基本知道这个镜像里有什么、要干什么,以及自己是否可以借鉴了。这个镜像的设计是 Docker 设计里最巧妙的地方之一,也是为什么大家都愿意认同,Solomon 要做的就是开发者体验(DX, Developer Experiences)。
FROM debian:jessie
MAINTAINER Hyper Developers <dev@hyper.sh>
RUN apt-get update &&\
apt-get install -y autoconf automake pkg-config dh-make cpio git \
libdevmapper-dev libsqlite3-dev libvirt-dev python-pip && \
pip install awscli && \
apt-get clean && rm -fr /var/lib/apt/lists/* /tmp/* /var/tmp/*
RUN curl -sL https://storage.googleapis.com/golang/go1.8.linux-amd64.tar.gz | tar -C /usr/local -zxf -
RUN useradd makedeb && mkdir -p ~makedeb/.aws && chown -R makedeb.makedeb ~makedeb && chmod og-rw ~makedeb/.aws
RUN mkdir -p /hypersrc/hyperd/../hyperstart &&\
cd /hypersrc/hyperd && git init && git remote add origin https://github.com/hyperhq/hyperd.git && \
cd /hypersrc/hyperstart && git init && git remote add origin https://github.com/hyperhq/hyperstart.git && \
chown makedeb.makedeb -R /hypersrc
ENV PATH /usr/local/go/bin:/usr/local/sbin:/usr/local/bin:/usr/sbin:/usr/bin:/sbin:/bin
ENV USER makedeb
ADD entrypoint.sh /
USER makedeb
WORKDIR /hypersrc
ENTRYPOINT ["/entrypoint.sh"]
一个规范的 Docker Image 或者脱胎于其中的 OCI Image,实际上就是一组元数据和一些层数据,这些层数据每个都是一个文件系统内容的打包,从某种意义上说,典型的 Live CD 的 OS,基本上可以理解成一个只读层加上一个可写层的 Docker Container 的 rootfs。在 Docker 这里,Union FS 可以说是 Great Again 了。
未来:现代化的镜像系统
然而,尽管 Docker Image (或者说 OCI Image)的设计蕴含了“完整的操作系统就是一个包”的优秀思想,又利用 Union FS 实现了“分层”这种既实现漂亮的开发者体验,又能节约时间空间的精巧设计,但随着时间的推移,还是暴露出来了一些问题。从去年(2019年)年初,OCI 社区中开始有人讨论下一代镜像格式的问题,这个热烈的讨论中,集中讨论了 OCIv1(实际也是 Docker 的)镜像格式的一些问题,Aleksa Sarai 也专门写了一篇博客来讨论这个话题,具体说,除了 tar 格式本身的标准化问题外,大家对当前的镜像的主要不满集中在:
- 内容冗余:不同层之间相同信息在传输和存储时都是冗余内容,在不读取内容的时候无法判断到这些冗余的存在;
- 无法并行:单一层是一个整体,对同一个层既无法并行传输,也不能并行提取;
- 无法进行小块数据的校验,只有完整的层下载完成之后,才能对整个层的数据做完整性校验;
- 其他一些问题:比如,跨层数据删除难以完美处理;
上述这些问题用一句话来总结就是“层是镜像的基本单位”,然而,镜像的数据的实际使用率是很低的,比如 Cern 的这篇论文中就提到,一般镜像只有6%的内容会被实际用到,这就产生了实质性的升级镜像数据结构,不再以层为基本单位的动力。
可见,下一代镜像的一个趋势就是打破层的结构来对这些只读进行更进一步的优化,是的,反应快的同学可能已经回想起了前面提到的 Live CD 里常用的 Squashfs,它可以根据读取的需要来解压相应的文件块来放入内存、供应用使用,这里是不是可以扩展一下,变成在需要的时候再去远端拉回镜像的内容来供应用使用呢——从文件的 Lazy decompress 到 Lazy Load,一步之遥,水到渠城。
是的,蚂蚁的镜像加速实践里就采取了这样的架构。在过去,庞大的镜像不仅让拉取过程变慢,而且如果这一过程同样风险重重,贡献了大半的 Pod 启动失败率,而今天,当我们把延迟加载的 rootfs 引入进来的时候,这些失败几乎被完全消灭掉了。在去年年末的第10届中国开源黑客松里,我们也演示了通过 virtio-fs 把这套系统对接到 Kata Containers 安全容器里的实现。
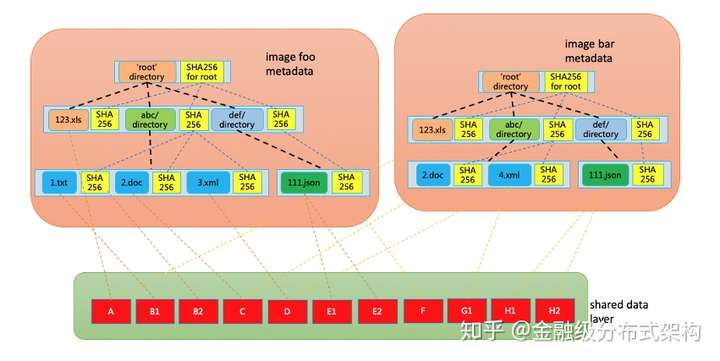
如图,类似 Squashfs,这种新的 Image 格式中,压缩数据块是基本单位,一个文件可以对应0到多个数据块,在数据块之外,引入了一些附加的元数据来做目录树到数据块的映射关系,从而可以在没有下载完整镜像数据的时候也给应用呈现完整的文件系统结构,并在发生具体读取的时候,根据索引,去获取相应的数据来提供给应用使用。这个镜像系统可以带来这些好处:
- 按需加载,启动时无需完全下载镜像,同时对加载的不完全镜像内容可以进行完整性校验,作为全链路可信的一个部分;
- 对于 runC 容器,通过 fuse 可以提供用户态解决方案,不依赖宿主机内核变动;
- 对于 Kata 这样的虚拟化容器,镜像数据直接送给 Pod 沙箱内部使用,不加载在宿主机上;
- 使用体验上和之前的 Docker Image 并没有显著不同,开发者体验不会变差;
而且,这套系统在设计之初,我们就发现,因为我们可以获取到应用文件数据访问模式的,而基于文件的一个好处是,即使镜像升级了,它的数据访问模式也是倾向于不太变化的,所以,我们可以利用应用文件数据的访问模式做一些文件预读之类的针对性操作。
可以看到,系统存储这个领域二十年来发生了一个螺旋式的进化,发生在 Live CD 上的进化,在容器这里也又来了一次,恍如隔世。目前,我们正在积极地参与 OCI Image v2 的标准推动,也正在把我们的参考实现和 DragonFly P2P 分发结合在一起,并成为 CNCF 的开源项目 Dragonfly 的一部分,希望在未来可以和 OCI 社区进一步互动,让我们的需求和优势成为社区规范的一部分,也让我们可以和社区保持一致、可平滑过渡,未来可以统一在 OCI-Image-v2 镜像之下。

You might not realise it yet, but you very likely want a better container image format than the ones currently available. How can I say this with such confidence? Because the current design of container images provides almost none of the properties that most people likely want from container images – even the properties you think you have aren’t omnipresent. The root cause of this problem is an unlikely and seemingly innocent suspect – tar
. But don’t let its appearance and familiarity fool you, tar
isn’t the format you might think it is.
This is the first of a series of articles I will be writing over the coming weeks, which will outline an improvement to the Open Container Initiative (OCI) image format in order to provide the properties that you want.
My first instinct was to title this article “Tar Considered Harmful”, but I had a feeling that the peanut gallery would cringe at such a title. However, this article is very much a foundational discussion of tar
and how it fundamentally fails in the use-case of container images (which will outline what we really want from a container image format). There have been some other articles that touch on the issues I go over here, but I hope I can provide a more cohesive insight into the issues with tar
. Then again, some folks believe that tar
is the best format for container images. I hope this first article will serve as a decent rebuttal.
I am currently working on a proof-of-concept of these ideas, and hopefully it will be ready soon for inclusions into umoci (a generic OCI image manipulation tool, which is being used by quite a few folks now-a-days for building and operating on container images).
But first, I guess we should make sure we’re on the same page on what container images actually are.
What [Container Images] Are Made Of
I hope that you’re already familiar with a concept of a container, so I’ll just give a one-line summary to make sure we’re clear I’m not talking about tupperware.
Containers are a virtualisation methodology where programs are run on top of a host kernel, but in a context where the filesystem (among other system resources) are virtualised. (To paraphrase Bryan Cantrill, “virtual” in this context is just a more diplomatic way of saying “lie”.)
Because the filesystem is virtualised, we need to have a different root filesystem than our host (just like a chroot
, which one could argue was the first instance of “something like a container”). Obviously a root filesystem is just a directory (or some union filesystem that looks like a directory at the end of the day). But then the follow-on question is “how are we going to distribute these directories?” – and that’s what a container image is.
Currently there are basically two models of container images:
- Layered container images. These are what most people think of when you say “container image”, and are made up of a series of layers which are stacked on top of each other during container execution (with a union filesystem or similar tricks). These layers are almost always
tar
archives, and is the beginning of the problem. Usually such images also contain several bits of metadata (usually JSON). - “Flat” container images. You can think of these as being more analogous to VMs. The most obvious examples of such container images are LXD’s images (which are effectively a single archive) and Singularity (which passes around a full filesystem image, which gets loopback mounted).
Most of the issues I will go over only really apply to layered container images. Due to the their design, “flat” container images have fewer problems (mainly because they aren’t interested in some of the features you get from layered images). In the case of LXD, their design actually handles some of these concerns anyway (in particular, “transfer de-duplication” isn’t necessary because they use binary deltas for updating images – and images are auto-updated by default on LXD).
This article will be focusing on OCI (Open Container Initiative) images, because that’s the standardised container image format (and I really hope it will get wider use if we can provide a clear advantage over other image formats). However, the same issues apply verbatim to Docker images – the OCI image format was based directly on the on-disk Docker format.
Just to make sure you get what an OCI image looks like, here is what it looks like after you’ve downloaded one (skopeo is a tool which translates images between formats, and also supports fetching images):
% skopeo copy docker://rust:latest oci:rust:latest
Getting image source signatures
Copying blob sha256:54f7e8ac135a5f502a6ee9537ef3d64b1cd2fa570dc0a40b4d3b6f7ac81e7486
43.22 MB / 43.22 MB [======================================================] 4s
Copying blob sha256:d6341e30912f12f56e18564a3b582853f65376766f5f9d641a68a724ed6db88f
10.24 MB / 10.24 MB [======================================================] 1s
Copying blob sha256:087a57faf9491b1b82a83e26bc8cc90c90c30e4a4d858b57ddd5b4c2c90095f6
4.14 MB / 4.14 MB [========================================================] 0s
Copying blob sha256:5d71636fb824265e30ff34bf20737c9cdc4f5af28b6bce86f08215c55b89bfab
47.74 MB / 47.74 MB [======================================================] 4s
Copying blob sha256:0c1db95989906f161007d8ef2a6ef6e0ec64bc15bf2c993fd002edbdfc7aa7df
203.34 MB / 203.34 MB [===================================================] 20s
Copying blob sha256:734ee16af2dd89c09a46ff408ffc44679aca2e1b8a10baec4febd9a7b6ac9778
218.11 MB / 218.11 MB [===================================================] 41s
Copying config sha256:af2dafa4b223aa1ab6ca6f6c35c5fce093254602cff4b2a8429850764d533b29
4.14 KB / 4.14 KB [========================================================] 0s
Writing manifest to image destination
Storing signatures
% tree rust/
rust/
├── blobs
│ └── sha256
│ ├── 087a57faf9491b1b82a83e26bc8cc90c90c30e4a4d858b57ddd5b4c2c90095f6
│ ├── 0c1db95989906f161007d8ef2a6ef6e0ec64bc15bf2c993fd002edbdfc7aa7df
│ ├── 2696f7292a958d02760e3a8964e554a3a6176fb7e04fc66be8760b3b05cbe65b
│ ├── 54f7e8ac135a5f502a6ee9537ef3d64b1cd2fa570dc0a40b4d3b6f7ac81e7486
│ ├── 5d71636fb824265e30ff34bf20737c9cdc4f5af28b6bce86f08215c55b89bfab
│ ├── 734ee16af2dd89c09a46ff408ffc44679aca2e1b8a10baec4febd9a7b6ac9778
│ ├── af2dafa4b223aa1ab6ca6f6c35c5fce093254602cff4b2a8429850764d533b29
│ └── d6341e30912f12f56e18564a3b582853f65376766f5f9d641a68a724ed6db88f
├── index.json
└── oci-layout
2 directories, 10 files
% find rust/ -type f | xargs file -z
rust/blobs/sha256/54f7e8ac135a5f502a6ee9537ef3d64b1cd2fa570dc0a40b4d3b6f7ac81e7486: POSIX tar archive (gzip compressed data)
rust/blobs/sha256/d6341e30912f12f56e18564a3b582853f65376766f5f9d641a68a724ed6db88f: POSIX tar archive (gzip compressed data)
rust/blobs/sha256/087a57faf9491b1b82a83e26bc8cc90c90c30e4a4d858b57ddd5b4c2c90095f6: POSIX tar archive (gzip compressed data)
rust/blobs/sha256/5d71636fb824265e30ff34bf20737c9cdc4f5af28b6bce86f08215c55b89bfab: POSIX tar archive (gzip compressed data)
rust/blobs/sha256/0c1db95989906f161007d8ef2a6ef6e0ec64bc15bf2c993fd002edbdfc7aa7df: POSIX tar archive (gzip compressed data)
rust/blobs/sha256/734ee16af2dd89c09a46ff408ffc44679aca2e1b8a10baec4febd9a7b6ac9778: POSIX tar archive (gzip compressed data)
rust/blobs/sha256/af2dafa4b223aa1ab6ca6f6c35c5fce093254602cff4b2a8429850764d533b29: JSON data
rust/blobs/sha256/2696f7292a958d02760e3a8964e554a3a6176fb7e04fc66be8760b3b05cbe65b: JSON data
rust/oci-layout: JSON data
rust/index.json: JSON data
J’accuse! There are our tar
archives, and you’ll notice that there’s one for each layer. There are also some JSON blobs, which aren’t really of interest to us here. There is also another important point to notice – OCI images use a content-addressable store as their backbone storage mechanism (index.json
is an “entry point” to the store – and is what contains the tags in most cases).
It should also be noted that OCI images all use “smart pointers” (that is, “pointers” which contain the content-addressable digest of the target as well as its media-type and size) which you can see in index.json
:
% jq '.manifests[0]' rust/index.json
{
"mediaType": "application/vnd.oci.image.manifest.v1+json",
"digest": "sha256:2696f7292a958d02760e3a8964e554a3a6176fb7e04fc66be8760b3b05cbe65b",
"size": 1146,
"annotations": {
"org.opencontainers.image.ref.name": "latest"
},
"platform": {
"architecture": "amd64",
"os": "linux"
}
}
These facts will become important later, when we talk about a new container image format that is built on top of the OCI content-addressable store (smart pointers and the ability to add new media-types will help us out).
As an aside, if you’ve ever wanted to know what the best container image format is, the short answer is basically “none of them”. The problem is that almost all of them have nice features that the others could really use, but because everyone wants to work on their own thing there’s much less cross-pollination than you’d like. Examples of such features include LXC’s templates, OCI’s content-addressability, or AppC’s dependencies.
What Has [Tar] Done For Us Lately?
tar
is a very old format, having been born within the original Unix source, and thus there is a lot of history within it. And, to no-one’s surprise, it’s a pretty ugly format in many ways. It simply doesn’t match what we need in a container image format, and I would argue it barely matches what most people today need in an archive format (though that’s out-of-scope for now).
Now, don’t misunderstand what I’m saying – my point here is not “it’s old, so it’s bad.” tar
is the obvious choice for an archive format, due to its long history and ubiquity, and writing a custom format with no justification would be a borderline reckless thing to do. However, tar
‘s history is important to understanding how it got to the state it’s in today. This section will be quite long-winded (there’s forty-something years of history to distil into a single blog post), but you can skip to the end.
This is not necessarily a new idea or argument, other folks have voiced similar concerns. I’m hoping that I can provide a cohesive overview of both tar
‘s generic issues as well as how its usage is even worse in the context of container images.
The full history lesson is a bit long (and probably something I’m not qualified to give) so I’ll just give you the highlights – if you’d like more in-depth information you can always take a look at pax(1)
, tar(5)
, star(5)
and the GNU tar internals documentation. This OCI PR discussion is a good example of how much back-and-forth can come about when discussing what an image specification “really means” when it says “tar
”.
Genesis
tar
first originated in Unix v7. Curiously, it was not the first archiving tool available for Unix. tar
was a successor to tp
(Unix v4), which itself was a successor to tap
(Unix v1). As a complete aside, this appears to be the reason why tar
accepts dash-less arguments (such as tar xvf
). Unix v1 didn’t have dashed argument flags like -xvf
(as far as I can tell from the man pages), and tar
appears to have been backwards-compatible with tp
(which was backwards-compatible with tap
). Therefore the most likely reason why tar
supports dash-less arguments is because some folks in the 70s wanted to be able to alias tap=tp tp=tar
and it’s stuck ever since. This should tell you what the primary theme of these history sections will be.
But that’s all besides the point. tar
was introduced in Unix v7, as a format for storing data on tape archives. It didn’t support compression. It didn’t even contain a magic header (so file foo.tar
couldn’t always tell you the file type). The design of the format was very simple, with fixed-length (512-byte) headers that contained all the information you might expect (file name, a single-byte “entry type”, length, mode, owner, modified time, and so on). If there was a non-zero length then it was followed by the contents of the file. This basic structure of tar
archives has been retained over the past 40 years.
To say that the format was strangely designed would be an understatement. First of all, all of the numerical values were stored in octal ASCII – which artificially limited the maximum entry size to about 8GB. In addition, symlinks (and hardlinks) were handled by storing the “link name” in the fixed-length header – resulting in each header containing 100 NUL bytes unless it was a symlink or hardlink. Obviously the pathname was restricted, but the restriction was exceptionally peculiar – rather than restricting the total pathname to 255 bytes, they restricted the basename
of the path to 100 bytes and the dirname
to 155 bytes (meaning that long dirnames
and long basenames
were both forbidden needlessly – and strangely tar
calls dirname
“prefix”). Curiously, the final 12 bytes of this 512-byte header remain unused in any standard to this day (it has been used by Solaris’s tar
as well as star
, but these are extensions).
Very soon, people started extending the original tar. The history of this is quite complicated, and tar
definitely went through all of the Unix wars (in a way, it’s a looking-glass for the history of Unix). Long before POSIX.1-1988 (which introduced ustar
) came around, there were a few competing implementations. Solaris’s tar
, FreeBSD’s bsdtar
, GNU’s tar
, and Jörg Schilling’s star
are the most notable. There was some cross-over between these different implementations, but eventually you ended up with a hodgepodge of different tar
-like archive formats (usually the same feature was re-implemented in different ways by different implementations). And, at the request of users, most of tar
implementations were forced to become somewhat interoperable with all of these other formats.
It’s important to keep in mind where tar
comes from to understand why its use is no longer reasonable.
The [Extension] Wars
Before we get into the flurry of extensions (and POSIX’s inability to contain them), I should probably explain how you might extend a tar
archive. I mentioned above that each tar
header contains a one-byte “entry type”. This is the primary way that extensions operate. All of the built-in entry types were ASCII decimals (with the exception of an ordinary file which could be represented either as a NUL byte or as '0'
). Before POSIX.1-1988, this was essentially a free-for-all, with various vendors coming up with their own custom header extensions as well as creating their own wacky entry types. It was definitely a fun time.
The most obvious things to extend should be pretty apparent – the limited size as well as pathname restrictions. GNU tar
partially fixed the size problem by storing size in “base-256”, and created special “long name” entry types that allowed you to have files (and links) with arbitrarily long pathnames. Sparse file support was added too, with varying degrees of support by other implementations (recall that interoperability requires everyone else to implement your special feature too).
Then the push for a “standard Unix for the masses” came along in the form of POSIX and the eventual release of the first edition of the standard, POSIX.1-1988. And tar
was included as part of this specification, with a new format called ustar
(Unix Standard TAR) that was meant to be the one format to rule them all. Unfortunately (like most things in POSIX) there was a need to placate every Unix vendor, and so the specification was incredibly generic and basic in terms of the features it defined. Most of the core properties of tar
were unchanged, though some quite important changes were made. For instance, POSIX.1-1988 requires all ustar
archives to set ustar\0
as the magic
field (and to add an empty header with just magic
set to ustar\0
at the beginning of the archive) so that tools like file
can actually reliably recognise ustar
archives (as I mentioned above, before this change, there was no reliable way of detecting whether something was a tar
archive).
Unfortunately, one of the largest problems with tar
compatibility was left woefully underspecified in POSIX.1-1988’s ustar
– how extensions should be handled between vendors. This was an issue that had caused lots of compatibility troubles in the past because implementations couldn’t recognise that the strange header they’re parsing was actually a foreign extension they didn’t support). The only “extension” handling that was provided by POSIX.1-1988 was that vendors could use any upper-case letter (all 26 of them) to store their own implementation-defined extensions and headers. After all, who would need more than 26 extensions – right?
Unsurprisingly, this didn’t help resolve the issue at all. GNU, Solaris, star
, and several others started using up this very limited namespace for a variety of their own extensions. As I mentioned above, file names and link targets were hideously restricted in length, and so GNU used L
and K
(not to mention their previous usage of N
in the old GNU format) for this purpose. And so the namespace became saturated with all of these different extensions, with people being worried about conflicts between different implementations – a rather odd example is that the “POSIX.1-2001 eXtended” format uses X
as an extension header, despite this header having been used by Solaris for a very long time. Another quite problematic conflict is that both GNU tar
and star
used S
to represent sparse files, but had slightly different semantics which usually ended brilliantly. So everyone went back to supporting everyone else’s extensions to keep users happy, and POSIX pretty much sat on their hands in this department until 2001.
Interestingly, as far as I can tell, we never actually used up all 26 extensions slots. But there were still a bunch of conflicts within the slots that were used (such as S
sparse file support). I guess you could argue this is a side-effect of the Birthday “Paradox” or we’re just really bad at sharing resources between different implementations.
PAX: A New [Standard]
In 2001, POSIX declared that enough was enough. It was clear that ustar
hadn’t solved the issues that they’d hoped to solve (vendor compatibility and modernising the Unix v7 tar
format). In addition, the tar
vs. cpio
war hadn’t fizzled out – and POSIX wanted to have One Format To Rule Them All™. So POSIX.1-2001 scrapped cpio
and ustar
and came up with a new format, called PAX (apparently “pax” is meant to to be a pun, since it means “peace” in Latin – and the intention of PAX was to bring peace between the tar
and cpio
camps).
PAX is effectively ustar
but with a series of extensions that they hoped would alleviate some of the issues that weren’t fixed by ustar
. While POSIX might refer to PAX as being a different format from tar
, when someone these days uses the word “tar” they usually are referring to PAX. The only thing PAX stole from cpio
is its lovely command-line argument design in the POSIX-defined tool pax
(which was meant to replace the need for tar
and cpio
– though of course tar
just ended up supporting PAX, cpio
is still alive and kicking, and almost nobody has even heard of pax
).
The primary extension was the addition of “pax Header Blocks”, which is a pair of new entry types that allow for key-value metadata to be applied for a given ustar
entry (x
applies it to the next entry, while g
applies the metadata to the entire archive and must appear at the start of the archive). The metadata is stored as the “file contents” of the entry, with each key-value mapping being stored as key=value
(separated by NUL bytes). A variety of keywords were defined as part of PAX, which deprecated older vendor extensions (examples include long names with path
and linkpath
, large file sizes with size
, as well as support for custom text encodings with hdrcharset
and charset
). Interestingly, pre-PAX there was no standard way to represent the atime
or ctime
of a file since the Unix v7 header only had a field for mtime
. PAX “resolved” this issue for the most part, though see the next section for more details.
Another interesting extension was to add an end-of-archive delimiter, which is two empty 512-byte headers (meaning all PAX-compliant tar
archives have a 1K blank footer).
For extensions they decided to create a much more fully-fledged extension system than existed in ustar
. Keeping with the theme of “uppercase ASCII is vendor space”, they allowed vendors to use keywords in the form <VENDOR>.<keyword>
(with <VENDOR>
being a vendor-specific name in all-caps). This opened the door to arbitrarily many vendor-specific extensions – with each vendor using their own namespace! This is nice, though as we’ll see in a minute, it did come with some downsides.
All-in-all, PAX was a fairly large improvement to tar
. They standardised some things vendors had been doing for a while, but unfortunately (like all POSIX standards) there were several things that were left under-specified. Extended attributes is the most obvious example, as well as how to handle new file-types (other than just doing it the old-fashioned ustar
way).
The [Extensions] Strike Back
With POSIX.1-2001 and PAX, surely we’re all done and there’s nothing left for vendors to extend, right? Oh my sweet summer child, if only that were the case. To cut the vendors some slack (especially Jörg Schilling’s star
, where most of the work on sane extensions has happened), PAX simply didn’t specify enough things to be usable as an archive format on modern Unix-like systems. So extensions were necessary, and this time folks weren’t limited to just 26 extension slots.
star
has an enormous number of extensions, many of which I won’t get into here because most of them are exceptionally niche and you probably aren’t interested. But there are a few important ones we should quickly discuss.
Extended attributes are an absolutely awful beast, and tar
makes it even harder to actually use them. First of all, not all Unix-like systems have the same ideas of what an extended attribute is (since it’s – surprise – not defined in POSIX and yet everyone has their own flavour of it). This automatically makes it ludicrously hard to support them in the first place, but then you get into how the support actually turned out – and that’s a whole different flavour of trash-fire.
There are five different extensions for storing them. The BSDs use LIBARCHIVE.xattr.<name>=<value>
and star
uses the very similar SCHILY.xattr.<name>=<value>
(though only libarchive
supports binary extended attributes using HTTP-style %
-encoding). Apple’s tar
is really out there and uses a special “resource file” with ._
prefixed to the basename of the file in question, which contains some Apple-specific magic that is used to represent extended attributes. AIX tar
uses the E
typeflag – because it was added during the pre-PAX days, as does Solaris (though of course, it’s done incompatibly). If you’re confused, don’t worry – so is everyone else.
Another related problem to extended attributes is “POSIX” (it was never in an actual standard) and NFSv4 ACLs. On Linux, NFSv4 ACLs are represented as extended attributes, which is a really fun time. I won’t get too far into ACLs, since you rarely run into them. But in short, star
has a lot of extensions for NFSv4 ACLs and POSIX.1e-2001 ACLs are fairly complicated, to say the least. Again, there are incompatibilities between different implementations.
There are some forms of extended metadata that most people forget exist, like chattr(1)
“file attributes”, which are not even included in most vendor implementations (star
uses SCHILY.fflags
). Yet again, this is another case of an extension that wasn’t widely supported (GNU tar
doesn’t support this metadata type at all, as far as I can tell).
Another problem that arose out of the current extension hell is that you can have files that use different extensions for the same tar
entry (not all extensions support everything you might want – so you need to mix-and-match for some cases). This massively increases the complexity of most tar
implementations (and some implementations like Go’s archive/tar
are still struggling with it).
I could go on with the countless extensions and problems that arise form them, but I’m sure I’m boring you by now. The key take-away is that these extensions have all resulted in the same interoperability issues as the past, and in quite a few cases vendors re-invent each others’ extensions (because they need them, POSIX doesn’t provide them, so they end up NIH-ing them).
Where Are We Today?
As a result, these days when you refer to tar
you are actually referring to a collection of different formats that have been re-implementing each others’ extensions slightly differently for decades. And while PAX, star
, BSD tar
, and GNU tar
are all mostly interoperable there are decades worth of legacy powering this whole ship.
Examples of where issues like this crop up are Go’s archive/tar
library that now has a deceptively simple-looking Format
attribute which allows you to forcefully select a tar
format to use (if you don’t explicitly use GNU or PAX then the atime
and ctime
will not be included in the archive). Furthermore, recent Go versions have changed the default output of archive/tar
in ways that are new readings of the PAX specification. To put it simply, tar
is what most implementations seem to support (which is usually PAX-plus-extensions) – and that’s not a really good bedrock to use for a new standard (as I mentioned before, even agreeing on “what is tar
” can be difficult).
I will admit that I enjoy using tools that were written long before I was born (since I’m actually a huge critic of almost all NIH projects), but you should ask whether you are reaching for a tool out of familiarity or because you earnestly believe it is the best tool for the job.
But What Practical Issues Are There?
All of this history might be interesting (well, to me at least), but it’s hardly a reason to not use a format right? Any old format will have similar growing pains, and given the ubiquity of tar
it seems fairly drastic to not use it just because it’s old. Unfortunately there are a whole host of practical problems with tar
for container images, which can be found by looking at what we might want in a theoretical container image format. Here is the list I have, and will be working through:
- Machine-independent representation.
- De-duplication (both transfer and storage).
- Parallelisable (both transfer and extraction).
- Sane handling of deleted files.
- Reproducible, with a canonical representation.
- Non-avalanching.
- Transparent.
For each of these, I will go into some depth what they mean and how tar
-based container images cannot provide them to a degree that is satisfactory. It might be a bit of a long ride, but I hope that this will help explain why tar
is fundamentally not a good match for this problem. It might be possible to modify tar
beyond recognition, but then the only benefit of tar
(it’s ubiquity) is lost because we’ve just created an incompatible variant of tar
. In fact (as we’ll see in a minute), container images already are incompatible variants of tar
when you look at how white-outs work!
Machine-Independent Representation
Specifically, it should be possible to create a container image on any given machine and it should work on any other machine. In fact, ideally you would hope that machine-specific configurations shouldn’t affect the container image’s creation and all machines should be able to equally use the image regardless of their machine-specific configuration. The latter statement is more general and is harder to get.
Arguably, this is something that tar
was designed for. And so it does quite well here – most machine-specific things (inode numbers, filesystem-specific layout information, and so on) are not embedded into tar
archives. Similarly, extraction of a tar
archive is the same regardless of filesystem.
So, we’re all good – right? Unfortunately no. While tar
does quite well here, you can run into a variety of issues very quickly.
First of all, tar
archive entries can be put in any order and it’s still semantically the same tar
archive. This is a problem for reproducibility but let’s deal with that later. In the context of machine-independence, the ordering of a tar
archive’s entries can be impacted by the filesystem used. In particular, the ordering of directory entries in readdir(3)
is dependent on how the filesystem stores directory entries. Many container image implementations sort them in user-space in an attempt to get around this problem, but most tar
implementations do not. Thus, to preserve ubiquity we must admit that tar
can result in this type of change based on host-specific conditions. Extraction is unaffected by this, but it harms reproducibility.
In addition, extended attributes (xattrs
) are a real pain. Their ordering in llistxattr(2)
is also completely filesystem-dependent and will affect how they are ordered in the tar
archive (not to mention there are several ways of representing them). There are several other problems with xattrs
, which I will expand on in reproducibility.
All-in-all though, tar
does pretty well here. Too bad this is the only section where that’s the case.
Lack of De-duplication
De-duplication is pretty important for container images, because without it we might as well be shipping around a rootfs archive for the entire image each time (this is actually want LXD does – though with a bit more care).
It should be noted that I’ve separated de-duplication into two forms, since there is a clear difference between not having to re-download bits that you already have (transfer de-duplication) and saving disk space when the image is on-disk and in-use (storage de-duplication). Ideally our format should help us with both problems, but different users care about one more than the other (depending on what they are optimising for).
To put it bluntly, tar
archives provide no standard method of de-duplication and in fact almost encourage duplication on every level (and the extensions that add de-duplication won’t help us). tar
archives have no internal de-duplication other than hard-links which are not really a form of de-duplication within our format because they require the on-disk image to be using hard-links.
What we’re really talking about here is how tar
layers operate with regards to de-duplication. And to be honest, tar
layer-based de-duplication is effectively useless outside of the FROM <foo>
flow of Dockerfiles. Updating a base image requires you to re-download the whole thing and store it entirely separately. If only a single byte in a single package has changed, that’s tough – you just have to re-download and store another 50MB. That’s just awful, and has resulted in a lot of folks moving to smaller container images (which is a mistake in my opinion – distributions serve a clear purpose and hacking away bits of a distribution image or switching to a niche distribution shouldn’t be done lightly).
In addition, there are many places where duplication is rampant:
- If you modify the metadata or bit-flip a large file in a lower layer, the next
tar
layer has to include the entire file contents.tar
doesn’t have metadata or partial-content entries. Solaris had an extension for it calledLF_META
, but see my above rant about extensions.star
also has a similar (but incompatible) extension using PAX’s keywords withSCHILY.tarfiletype=meta
, and the same rant applies. - If you delete a file, then a “white-out” needs to be stored in the next layer (which is effectively a tombstone) – meaning that removing a file increases the size of our image. As an aside, this tombstone actually means that standard
tar
implementation will not be able to correctly extract a container image (we’ve already forked from standardtar
). A very fun restriction added by these tombstones is that you cannot have a container image that has a file containing a.wh.
prefix. I will go into more detail about white-outs in a later section. - If you create a hardlink to an existing file in a previous layer, in order for the new layer’s
tar
archive to be valid you need to copy the original file into the newtar
archive as well as add the hardlink entry (tar
archives have hardlink entries which just store the target of the link). This is fairly expensive duplication (especially if the file is large) and can’t really be fixed without generating archives that are no longer valid and self-contained. Hardlinks are also a pretty large pain in thetar
format anyway, but I won’t get into that much here.
And while you do get layer de-duplication because layers are content-addressable, the layers themselves are so fragile (a single bit-flip makes the entire layer hash different) that you end up with very little practical de-duplication (of transfer and storage).
Lack of Parallelisable Operations
Given that our machines have the ability to multi-task, it’d be nice if we weren’t bottle-necked on many image operations. In particular, transfer and extraction (taking the image from it’s OCI representation and actually putting into a storage driver) are very important to parallelise if possible.
A single tar
archive cannot be extracted in parallel without a single linear pass (to figure out where the headers start) since tar
archive entries are header-followed-by-content based. Adding an index might help with this, but requires adding more out-of-spec things to our tar
-like format. There are some other tar
forks that have indexes, but as you’ll see in a second we’d need something a bit more complicated.
But what about extracting layers in parallel? I’m sure I’m not the only person who has been frustrated that a lot of the time spent on getting a cold-start container to run is in extracting the image. There are a few problems with extracting tar
layers in parallel (though it actually could be possible to do, it would just be quite difficult without more extensions). Since two layers can contain the same file but with different contents (which means that the file is extracted twice), and you have “white-outs” to deal with (which means that the file is extracted and immediately deleted) you can’t just extract them all concurrently. You could be more clever about it by extracting them in parallel and making sure that earlier layers don’t overwrite later ones. But you’re still subject to races (which would decide whether or not you extract the same file more than once) as well as making extraction code quite complicated (figuring out whether two non-existent paths refer to the same file would be a “fun” exercise, as well as dealing with hard-links and the like).
The obvious solution would be to add an index on the whole-image level which tells you what paths are present in each archive (and where their header offset is). There is a slight problem with using the header offset – the PAX extension headers (x
and g
) can scattered throughout the archive, and you need to know their values when interpreting a tar
header. Which means you have to store the whole header once parsed, and then you can use the content offset to extract everything in parallel (since you know which layer has the latest copy of the file). Unfortunately we’ve just out-sourced the header information to a separate index, and the archives are now just being used as content stores – which means we’ve invented our own format that uses a stunted form of tar
. All of this work and gymnastics for no good reason.
In addition, most container images use compressed tar
archives. A compressed archive cannot be seeked without extracting everything before it, making partial extraction (or other such partial operations) needlessly expensive. Duplicity hit this problem, and the only way of solving it is to make compression happen underneath the archive format (not above it, as is the case with tar+gzip
).
Insane Handling of Deleted Files (White-outs are Awful)
This issue is an overlapping of a few other issues such as de-duplication and parallelisation, but is specifically focused on deleted files and white-outs because they deserve extra-special attention.
Because it’s possible for a file to be deleted in a layer, it’s necessary to be able to represent this in a tar
archive. And immediately we’ve hit a barrier – tar
doesn’t support this concept at all. So it’s necessary to have some kind of extension for it.
In order to support deleting files in layers, the OCI image format adopted AUFS’s on-disk format (the reason for this is historical and is because Docker baked the AUFS on-disk format into their image format since it was the only storage driver they supported originally, and this has been carried into the OCI as legacy). Deleted files (and directories) are represented as an empty regular file (known as a “white-out”) with .wh.
prepended to their basename
. Aside from being incompatible with other tar
implementations (which will just extract the weird .wh.
file without knowing what it is) it also means that you cannot include a real file with a prefix of .wh.
inside any OCI image. Personally, I think embedding AUFS’s format was a fairly big mistake but we’re stuck with it for now. There are other ways of dealing with deleted files, but they all have similar problems with interoperability:
- Using
SCHILY.filetype=white-out
is possibly the best solution, since it’s already used by BSD and exists specifically to represent opaque directory entries created through BSD’smount_unionfs
. However it is astar
-only extension, and arguably would be somewhat lying about the source of the filesystem being from a BSDmount_unionfs
(though it would probably interoperate just as well withstar
). - Using a special entry type that we create ourselves. If an implementation sees our white-out entry type, they will at least have an opportunity to fail loudly (which is somewhat better than the fail-silent
.wh.
approach we have right now). But obviously most implementations won’t support our special white-out entry type, breaking interoperability. - Having an external deleted file list. This is nice because it doesn’t require touching the
tar
format, but it comes with the downside that the archives no longer fully describe the root filesystem (and users have to be aware of this because it no longer is just “good oldtar
”). If we have to supplementtar
to make it work, why still usetar
? - Copying the representation that Linux’s
overlayfs
uses, which is to use device number{0,0}
for non-directories and thexattr
overlay.opaque=y
for directories. The main problem with this is that it is repeating the AUFS mistake again by baking a particular overlay filesystem’s representation of white-outs into a format – as a result it won’t be interoperable. Not to mention that if you wanted to store a realoverlayfs
directory inside a container image you wouldn’t be able to (because on extraction there would be no way of telling if the white-outs are meant to be inside the image or are the image’s own white-outs). In addition,overlayfs
has changed their white-out format in the past, so baking it into our format seems like a bad idea. - Creating our own fake
xattr
(likeopencontainers.whiteout=y
) to represent all white-out files. This is potentially better than copyingoverlayfs
, as it means we don’t need to worry about not being able to representoverlayfs
directories inside container images. It also doesn’t conflict with anything, because we invented it. And (on Linux at least), thexattr
namespaces are quite restrictive to write to and I don’t think you could actually setopencontainers.
xattrs on any Linux filesystem (though on other operating systems this might be possible – which would lead to issues of not being able to store any universal filesystem structure). The main downside is that we are explicitly removing any chance of interoperability without convincing othertar
implementations to implement our weird format.
All of these are fairly disappointing solutions (though it is nice that star
has something we could re-use that is at least somewhat interoperable). This is a direct result of trying to have a layered format built with another format that wasn’t designed for layering. In addition, layering causes fun problems because the image history is contained in the image. Embedding the history of an image in every image has caused some security concerns in the past related to having build-time secrets that would be included in layers and attempting to redact them by deleting them didn’t remove them from the previous layers (something that is somewhat of a restriction made by using tar
layers, but is also more of a workflow issue).
Lack of Reproducibility and Canonical Representation
Reproducible builds have gotten quite a lot of hard work put into them in the past few years. These days, a vast majority of the packages available in distributions are built bit-for-bit reproducibly – which is an astonishing achievement (and allows for far more independent verification of binaries). It would be fairly self-defeating if the packaging format we use for containers wasn’t also reproducible. Not to mention that reproducible images would mean that two image generators that have never communicated could benefit from de-duplication. You could reproducibly create a distribution image yourself (using the distribution’s build scripts and sources), and still be able to de-duplicate with it! A canonical representation is very important in order to make sure that all image generators will always produce reproducible representations of the image (any lee-way will allow for dissonance). Not to mention that you could now verify distribution images in more ways than currently available (such as adding your own extra verifications during image build and verifying that the final image is identical to the distribution one).
Due to a large variety of reasons, tar
archives are practically impossible to reproduce effectively. While there is no technical reason why they are hard to reproduce, there are a myriad of complications that make it difficult to reproducibly create the same archive. There are projects which attempt to solve this problem, but the fundamental issue remains (and tar-split
only allows you to take an archive and make it look like another version of itself if you have the pre-generated metadata).
One of the most obvious problems (as mentioned in the machine-independent section) is that you can re-order archive entries without issue. This results in trivially different representations, and without a canonical representation they’re all as good as one another (most implementations end up storing them in the order given by the filesystem).
The other really obvious problem is that different extensions overlap significantly, resulting in there being many different ways of representing (with different extensions) things not available with the base tar
format. Examples include long path names, or new file types. Compounded with no canonical format (and that different tar
generators and consumers having differing levels of support for mixed extensions in the same archive), you end up with a real mess and the same filesystem having many different representations.
Extended attributes are a really bad problem, on many levels (to the point where I could make a whole blog post just about that). As I mentioned in the history of tar
, there are five different extensions for storing them. This means that an implementation could use any of them and still be a valid tar
archive – furthering the canonical representation problems.
Then you have how languages treat xattrs
. Since they’re basically a key-value store for metadata almost every library developer thinks they should be stored in hash tables. This means that their iteration order is randomised. Which means that the output archive has random xattr
order and thus is not reproducible and has no canonical format. Many languages also incorrectly assume that xattrs
can only contain valid UTF-8 (or ASCII) strings – this is also false, they can contain arbitrary binary data. I have yet to see a tool that handles this correctly. Also empty xattrs
are entirely valid, but PAX doesn’t allow them – so there are valid filesystems that cannot be represented with tar
(aside from the .wh.
problem I outlined earlier). How awesome is that!
But my favourite thing is that a given tar
implementation can start producing different archives between versions, for any variety of reasons. With Go, there were a series of releases where each one changed the default output of the built-in archive/tar
library. It got so bad I had to add regression tests for the language in umoci. And all of this is possible because there is no defined canonical representation of a tar
archive and so library developers feel free (quite rightly) that they can change the default output – it’s entirely our own fault we’re depending on it not to change (there was even a proposal to randomise the output of archive/tar
).
Avalanching
I’ve borrowed this term-of-art from cryptography. In this context, it means that a small change in an image results in a disproportionately large change such that we need to re-download or store much more data. This is slightly different from de-duplication in that it’s about the way the format handles small changes rather than how we handle similar data throughout all images (though a single solution can solve both issues).
tar
layers are avalanching by nature, because any change in a layer results in us needing to download the whole thing all over again. I’m not sure it’s necessary to elaborate this point, since I went over it in the de-duplication section.
Lack of Transparency
This last one is a fairly nebulous goal, and is one that will require quite a bit of thought. Effectively the problem is that currently almost all techniques for finding security vulnerabilities is to scan the filesystem. But distributions already know what packages they have (and what security vulnerabilities were fixed in those package versions). So why are we duplicating work – why can’t you just have a verifiable manifest of the packages in an image?
In the Open Build Service we have this, though it’s only used internally so that OBS knows when to re-build a container image (if any of the packages in the image are updated in the dependency tree). However, this information would be useful to more than just distribution folks – the security status of packages is something that distributions and distributions alone know for sure.
In this case, tar
doesn’t make things easier or harder for us. Transparency needs to be added as a manifest on top (though tar
archives might make verification of the manifest harder, since they need to be extracted in-memory and also the format is opaque to the OCI image archives). With a less opaque format, it might be possible to make it easier to verify that a particular package is verbatim present and that the manifest is complete.
I’ll be honest, my current ideas for how to solve this issue are quite primordial (compared to my solutions for the other issues I’ve listed). I believe my new format idea could help make this easier, but it will still require a fair bit of work (ideally a Merkle tree would allow us to combine the filesystem tree of packages and verify that a package is present fairly trivially, but doing so would compromise the canonical representation goal). I’m still thinking on how this particular issue can be solved.
How Do We Get It?
I’m afraid to find that out, you’ll need to wait until the next instalment. I hope to get it complete in a few weeks (I was hoping to have a PoC available with the next instalment but that’s just a silly goal at this point).
If you want a taste though, the general idea is that we can resolve most of the issues I’ve listed and gain most of the properties we want by creating our own format that is built on top of the OCI content-addressable store, and is a Merkle tree with content-defined chunking of file contents. The basic idea is very similar to backup tools like restic or borgbackup. Since OCI has smart pointers, we can define a few new media-types, and then our new format would be completely transparent to OCI image tools (as opposed to opaque tar
archives).
But you’ll learn all about that next time. Thanks for reading, and happy hacking!
Unless otherwise stated, all of the opinions in the above post are solely my own and do not necessary represent the views of anyone else. This post is released under the Creative Commons BY-SA 4.0 license.
Want to keep up to date with my posts?
You can subscribe to the Atom Feed.